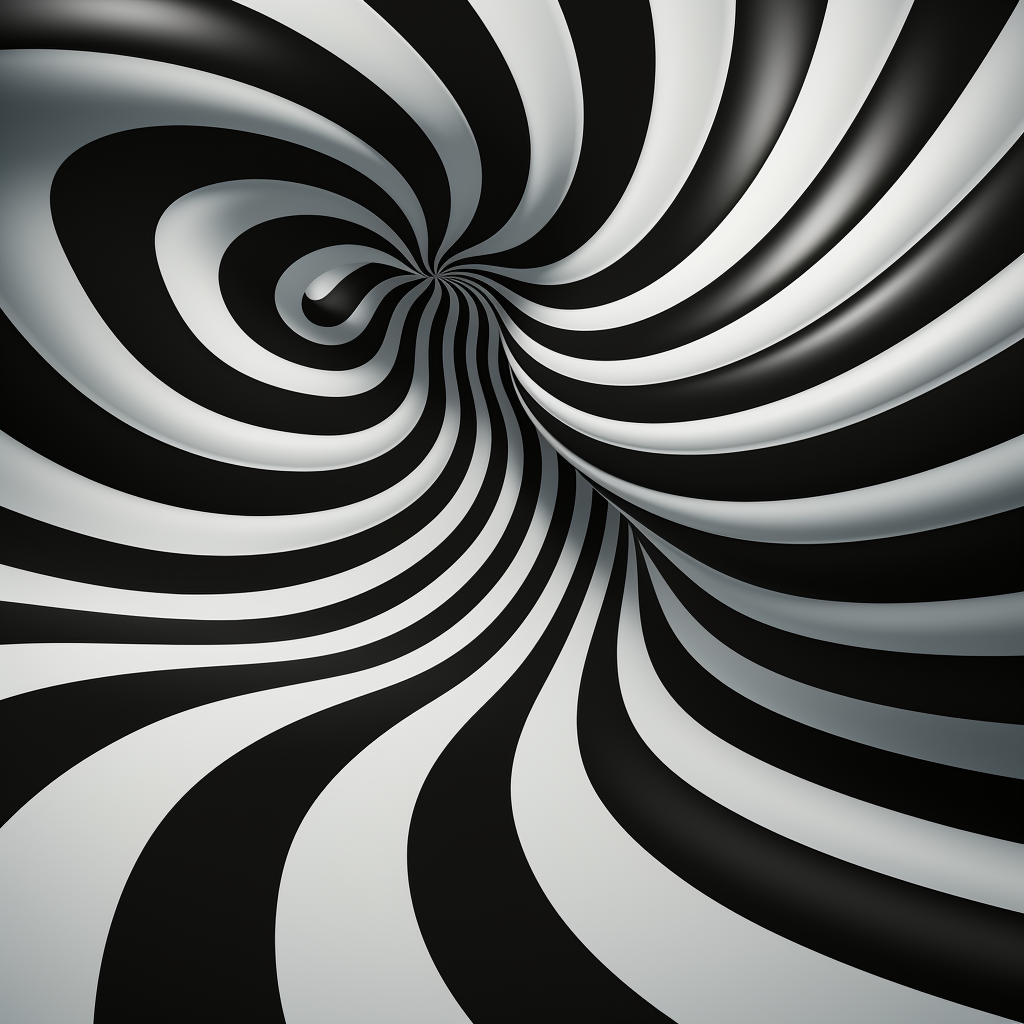
How does the Doppler effect work?
The Doppler Effect is a fundamental principle in physics that describes how the frequency of a wave changes based on the relative motion between the observer and the source of the wave. Named after Christian Doppler, an Austrian physicist who proposed the concept in 1842, the Doppler Effect applies to any type of wave, including sound, light, and other electromagnetic waves.
Let’s begin by discussing the Doppler Effect in the context of sound waves, which is perhaps the most intuitive way to understand the principle.
Doppler Effect with Sound Waves
Imagine you are standing by the side of a road, and a car with a continuously honking horn is approaching you. As the car approaches, you notice the sound of the horn seems to have a higher pitch. However, as the car passes you and moves away, the pitch of the horn appears to decrease. This change in the perceived frequency (and thus the pitch) of the sound due to the relative motion between you (the observer) and the car (the source of the sound) is a direct manifestation of the Doppler Effect.
When the car is approaching, each successive wave crest of the sound has less distance to cover to reach you, so these wave crests hit your ear more frequently – this increases the frequency of the sound waves, resulting in a higher pitch. Conversely, when the car is moving away, each wave crest has a further distance to cover to reach you, so these wave crests hit your ear less frequently – this decreases the frequency, and you perceive a lower pitch.
Doppler Effect with Electromagnetic Waves
The Doppler Effect is also applicable to light and other electromagnetic waves. The change in frequency (and thus energy) of light due to the Doppler Effect results in a change in the color of the light. If an object is moving towards the observer, the frequency of the light increases, and the light appears more blue, an effect known as blueshift. If the object is moving away from the observer, the frequency of the light decreases, and the light appears more red, an effect known as redshift.
In astronomy, the Doppler Effect is a powerful tool used to measure the velocity of stars and galaxies relative to the observer on Earth. For example, Edwin Hubble, in the 1920s, observed a redshift in the light from distant galaxies, which indicated that these galaxies were moving away from us. This was the first empirical evidence supporting the theory of an expanding universe.
Mathematical Description
The Doppler Effect can be mathematically represented using the following formula:
f’ = f * (v + vo) / (v + vs)
where:
- f’ is the observed frequency,
- f is the source frequency,
- v is the speed of the wave (sound speed or light speed),
- vo is the speed of the observer relative to the medium (positive if the observer is moving towards the source),
- vs is the speed of the source relative to the medium (positive if the source is moving away from the observer).
Note that this formula assumes that the speeds of the source and the observer are much less than the speed of the wave, which is a good approximation for most practical scenarios involving sound waves and low-speed objects.
Other Applications
Besides acoustics and astronomy, the Doppler Effect has various other applications, including radar systems, medical imaging (Doppler ultrasound to monitor blood flow), velocity measurement tools (Doppler radar guns used in traffic control), and satellite communication.
In conclusion, the Doppler Effect is a fundamental principle that describes the change in the frequency of a wave based on the relative motion between the observer and the source of the wave. It has widespread applications across different fields, from understanding the universe’s expansion to designing medical imaging devices and radar systems.
Doppler Effect in Special Relativity
The classical Doppler effect described above is a good approximation when the speeds involved are much less than the speed of light. However, when objects are moving at speeds close to the speed of light, the Doppler effect must be understood in the context of Einstein’s special theory of relativity, which takes into account the finite speed of light and the relativistic effects of time dilation and length contraction.
In special relativity, the formula for the Doppler effect (for light) becomes more complex, depending on whether the source and the observer are moving towards each other or away from each other, and whether the motion is along the line of sight or perpendicular to it. The relativistic Doppler effect causes shifts in the frequency (and thus the color) of the light and is a crucial tool in modern astrophysics and cosmology.
Doppler Effect in General Relativity
In the context of general relativity, which describes gravity as a curvature of spacetime caused by mass and energy, the Doppler effect has even more profound implications. For instance, the gravitational redshift is a phenomenon where light or other electromagnetic radiation from a massive object (like a star or a black hole) is redshifted due to the gravitational field of the object. This is a direct consequence of the Doppler effect and the curvature of spacetime, and it has been confirmed by numerous experiments.
Doppler Broadening
In atomic physics and quantum mechanics, the Doppler effect also leads to a phenomenon known as Doppler broadening, where the spectral lines of atoms or molecules are broadened due to the thermal motion of the particles. This is because the thermal motion causes a distribution of velocities along the line of sight, and each velocity contributes a different Doppler shift, leading to a broadening of the spectral line. Doppler broadening is a fundamental aspect of spectroscopy and is used to measure temperatures in various contexts, from stellar atmospheres to experimental plasma physics.
In summary, the Doppler effect is a cornerstone of wave phenomena, underpinning our understanding of sound, light, and other wave-based interactions. Its manifestations run the gamut from the everyday – the changing pitch of a passing vehicle – to the cosmic, aiding in the measurement of distant celestial bodies’ velocity. It also enables technologies like radar and medical imaging, making it not only scientifically fascinating but practically indispensable. It is a testament to how the observation of simple, everyday phenomena can lead to profound insights about the universe and its workings.