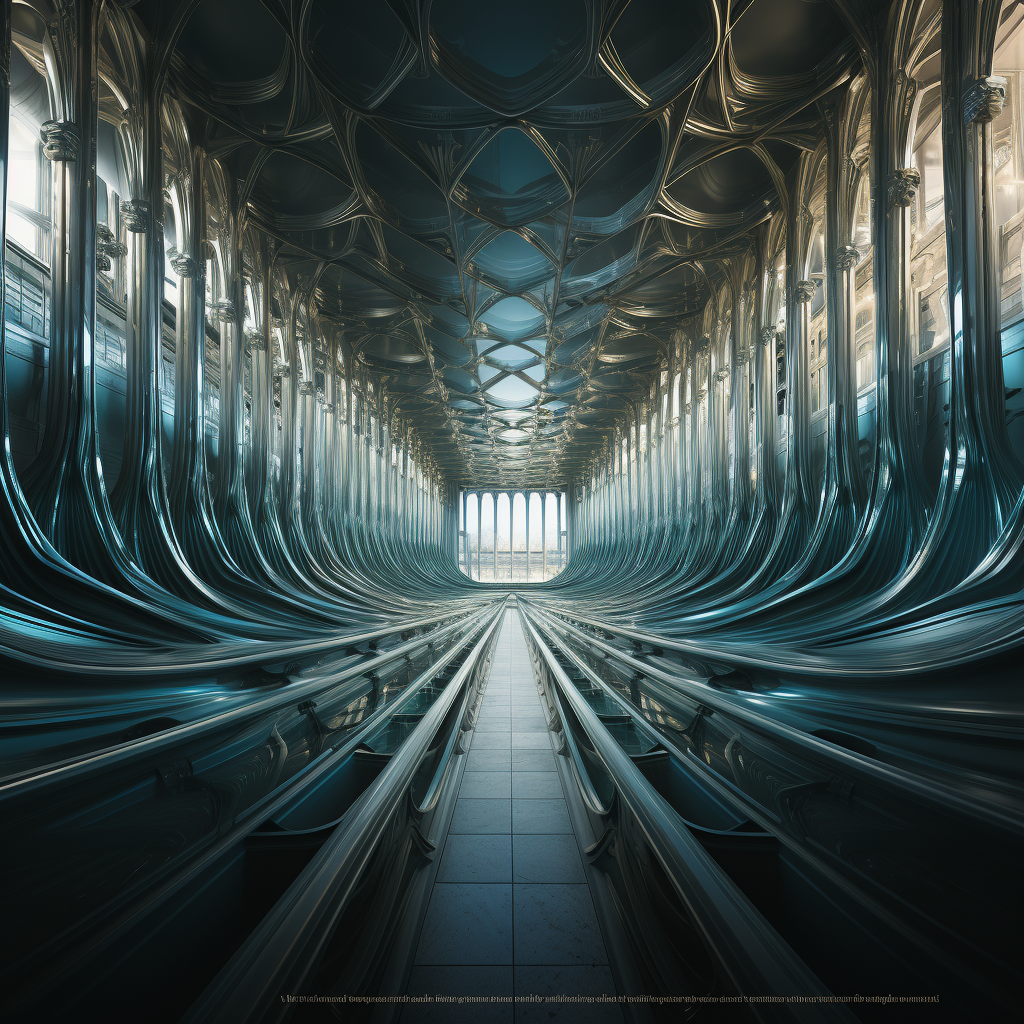
What are the principles of superconductivity and superfluidity?
Superconductivity and superfluidity are two phenomena that result from quantum mechanics and are observed when matter is cooled to extremely low temperatures. Both are macroscopic quantum phenomena that defy the behaviors we typically see in the everyday world, presenting intriguing insights into the nature of matter at its most fundamental levels.
Superconductivity
Superconductivity is a quantum mechanical phenomenon where a material, when cooled below a certain temperature known as the critical temperature, can conduct electric current with zero electrical resistance. This means that a superconductor can carry a current indefinitely without any loss of energy. This extraordinary property was first discovered by Heike Kamerlingh Onnes in 1911 when he observed mercury becoming superconducting at temperatures below 4.2 Kelvin.
Two important concepts underpin superconductivity:
Cooper Pairs
Leon Cooper proposed a theory in 1956 to explain why electrons, which should repel each other due to their like charges, can form pairs (Cooper pairs) that move through the lattice structure of a superconductor without scattering off impurities or lattice vibrations (phonons), thus not experiencing resistance. The formation of Cooper pairs is a result of a slight attractive interaction mediated by phonons. This attraction is stronger at lower temperatures, allowing for the formation of these pairs.
BCS Theory (Bardeen–Cooper–Schrieffer Theory)
The BCS theory, developed in 1957 by John Bardeen, Leon Cooper, and John Robert Schrieffer, explains how electrons form Cooper pairs and how these pairs lead to superconductivity. At low temperatures, Cooper pairs move through the superconducting material without resistance because the energy required to break them apart (and hence scatter them) is larger than the thermal energy available in the system.
A unique feature of superconductors is the Meissner effect, where a superconductor in the presence of an external magnetic field will expel the field from its interior. This phenomenon, which can cause a magnet to levitate above a superconducting material, is a direct result of the perfect diamagnetism of superconductors.
Superconductors have widespread applications, including in MRI machines, magnetic levitation trains, and particle accelerators. However, their use is often limited by the need for extremely low temperatures. The discovery of high-temperature superconductors (those that become superconducting at higher, albeit still quite cold, temperatures) has opened new possibilities, but the mechanism behind high-temperature superconductivity remains one of the biggest unsolved problems in physics.
Superfluidity
Superfluidity, like superconductivity, is a state of matter that arises at extremely low temperatures, in this case for certain liquids. A superfluid, such as helium-4 at temperatures below 2.17 Kelvin (the lambda point), flows without friction, exhibiting zero viscosity. This allows a superfluid to display strange behaviors, such as creeping up the walls of a container and dripping down the outside.
Superfluidity was first discovered in liquid helium by Pyotr Kapitsa, John F. Allen, and Don Misener in 1937. It’s a quantum mechanical effect that occurs when the matter in a system is condensed into the lowest energy state, or ground state. At such low temperatures, the quantum nature of the liquid becomes apparent at macroscopic scales. In essence, the particles behave more like a single entity rather than individual particles, allowing the superfluid to flow without resistance.
Superfluid helium-4, in particular, is described by a theoretical framework known as Bose-Einstein Condensation. This occurs with particles known as bosons, which can occupy the same quantum state, condensing into a single quantum state at low temperatures. This effect is directly responsible for the phenomenon of superfluidity.
Superfluidity has interesting and sometimes counterintuitive consequences. One of them is the phenomenon of “quantized vortices.” These are tiny whirlpools that can form in a superfluid, and the speed at which they rotate is quantized, meaning it can only take certain discrete values. This is a direct consequence of the wave-like nature of particles in the superfluid state.
Although superfluidity might seem like an esoteric concept, it has played a fundamental role in our understanding of quantum mechanics and its implications in the macroscopic world. It also has potential applications in precision measurement devices, quantum computing, and understanding neutron stars.
Both superconductivity and superfluidity are intriguing examples of quantum phenomena on macroscopic scales. They challenge our common sense with their remarkable properties and, at the same time, provide fascinating opportunities for technological innovation and fundamental research into the nature of matter and the universe.